Date | Presenter | Title/ about 15 minutes, < 20 minutes |
24-03-2025 | 1.YangNS | Geneious prime usage |
31-03-2025 | 2. ChenX | 1. Active in vivo translocation of the Methanosarcina mazei Gö1 Casposon. Nucleic Acids Res. 2023 2. Casposase structure and the mechanistic link between DNA transposition and spacer acquisition by CRISPR-Cas eLife 9:e50004. |
07-04-2025 | 3. Addy | Transposition of Mutator-like transposable elements (MULEs) resembles hAT and Transib elements and V(D)J recombination-2017-NAR |
21-04-2025 | 4. Wijdan | A Naturally Active Spy Transposon Discovered from the Insect Genome of Colletes gigas as a Promising Novel Gene Transfer Tool-2024-ADS |
28-04-2025 | 5. Ahmed | Characterizing Mobile Element Insertions in 5,675 Genomes |
6-5-2025 | 6. XXxing | All-RNA-mediated targeted gene integration in mammalian cells with rationally engineered R2 retrotransposons-cell |
19-05-2025 | 7. XianKL | Cas9-induced targeted integration of large DNA payloads in primary human T cells via homology-mediated end-joining DNA repair-2024-nbe |
2-6-2025 | 8. LiMY | Transposase-assisted target-site integration for efficient plant genome engineering-2024-nature |
9-06-2025 | 9. WanQ | Transposase N-terminal phosphorylation and asymmetric transposon ends inhibit piggyBac transposition in mammalian cells-NAR-2022 |
16-06-2025 | 1. Phiri | Genomic Analysis Revealed a Convergent Evolution of LINE-1 in Coat Color_ A Case Study in Water Buffaloes (Bubalus bubalis)-MBE-2021 |
23-06-2025 | 2. XinChen | Megabase-scale human genome rearrangement with programmable bridge recombinases-2025-preprint |
30-06-2025 | 3. Addy | Functional characterization of the active Mutator- like transposable element, Muta1 from the mosquito Aedes aegypti-mobile DNA-2017 |
4. Wijdan | Transposase expression, element abundance, element size, and DNA repair determine the mobility and heritability of PIF-Pong-Harbinger transposable elements-2023-front-cell-and-biology | |
5. Ahmed | An Alu insertion map of the Indian population: identification and analysis in 1021 genomes of the IndiGen project. NAR Genom Bioinform. 2022 | |
6. XXxing | 2025-nature-Reprogramming site-specific retrotransposon activity to new DNA sites | |
7. XianKL | ||
8. LiMY | ||
9. WanQ | ||
10. NSYang | ||
Protein Mutational Effect Predictor (ProMEP) |
研究进展
TnLabDatabase
Database developed by TnLab
pigRIPdb: pig retrotransposon insertion polymorphism database
TnpBMEdb: database of TnpB associated mobile elements
IscBMEdb: database of IscB associated mobile elements
rabbitRIPdb: rabbit retrotransposon insertion polymorphism database
sheepRIPdb: sheep retrotransposon insertion polymorphism database
goatRIPdb: goat retrotransposon insertion polymorphism database
auDNATndb: Autonomous DNA Transposons database (auDNATndb)
Programs developed by TnLab
TypeSINE: program for genotyping SINE RIP in livestock (mammal)
MEboundary: program for automated definition of TnpB and IscB associated mobile elements
转座酶及转座子分类
转座子(Transposon),也称为跳跃基因或转座元件,是一类可以在基因组中移动的DNA序列。它们能够从基因组的一个位置“跳跃”到另一个位置,这种现象称为转座。转座子广泛分布于原核和真核生物基因组中,曾被认为是基因组中的垃圾DNA。转座子的发现颠覆了传统上认为基因组是静态的观点,揭示了基因组的动态性。
转座子的分类
- DNA转座子(Class I):
- DNA转座子存在多种转座子机制,可分为:
- 1.剪切-粘贴型DNA转座子/Cut-and-Paste,例如: Ac/Ds, Tc1/mariner, PiggyBac
- 2. 复制-粘贴型DNA转座子/Copy-out-paste-in,例如:IS1,IS3,IS481,IS1595, IS21、IS30、IS256、IS110/IS1111、ISLre2、ISL3等
- 3. 滚环转座子/rolling-circle,例如:IS91 和 IS1294
- 4. 剥离粘贴转座子Peel and Paste,例如:IS200,
- 5. 自我合成转座子/self-synthesizing,例如:Polintons, Maverick
- 逆转录转座子(Class II):
- RNA转座子又称逆转录转座子,转座过程中需要RNA介导。
- 通过“复制-粘贴”机制移动,即转座子被转录成RNA,然后通过逆转录酶生成DNA拷贝,再插入到基因组的新位置。
- 依赖逆转录酶(reverse transcriptase)。
- 例子:真核生物中的LINEs(长散在核元件)和SINEs(短散在核元件)。
转座子的功能与影响
- 基因组进化:
- 转座子可以引起基因突变、基因重排和基因组扩增,推动基因组进化。
- 它们可能携带额外的基因片段,促进新基因的形成。
- 基因调控:
- 转座子插入到基因附近或内部时,可能影响基因的表达。
- 它们可以作为启动子、增强子或沉默子,调控邻近基因的活性。
- 疾病关联:
- 转座子的异常活动可能与某些疾病有关,例如癌症或遗传性疾病。
- 插入到关键基因中可能导致基因功能丧失或异常。
- 生物技术应用:
- 转座子被用作基因工程工具,例如在基因敲入、基因敲除和基因标记中。
研究意义
转座子的转座特性使其能够横向传播,打破了人们对生命进化过程中遗传物质垂直传递方式的传统认知,使不同物种间遗传物质传递成为可能。此外,转座能够产生丰富的基因组结构变异,增加生物基因组的多样性和可塑性,并可能引起生物的表型变异,进而可能会对生物的适应性和进化产生影响。研究表明转座子是驱动生物进化创新的重要因素。研究基因组上转座子的起源进化、转座子介导的结构变异及其对基因组进化和表型变异的影响,能够加深转座子在生命进化中的作用的理解和认识。另外,转座子的转座特性使其具有介导大片段基因转移的能力,在人类基因治疗和转基因生物育种也得到广泛应用。
总之,转座子的研究对理解基因组结构、功能和进化具有重要意义。它们不仅是基因组的重要组成部分,还为生物技术的发展提供了有力工具。
TnpB
TnpB 是一种与转座子相关的小分子靶向核酸酶,研究表明三类转座子(IS605, IS607, IS1341)含有TnpB。它是 RNA引导的核酸酶,在细菌和古菌中广泛存在。近年来,TnpB 因其与 CRISPR-Cas 系统的相似性而受到关注,被认为是 CRISPR-Cas 系统的进化前体之一。
TnpB 的功能
- 转座子移动的辅助蛋白:
- TnpB 是 IS200/IS605 转座子家族中的一种蛋白质,参与转座子的移动过程。
- 它与转座酶(TnpA)协同作用,帮助转座子完成“剪切-粘贴”机制。
- RNA引导的DNA切割:
- TnpB 通过结合一段小的 RNA(称为 ωRNA 或 transposon-encoded RNA)来识别目标 DNA 序列。
- 它能够切割目标 DNA,类似于 CRISPR-Cas 系统中的 Cas 蛋白。
- 基因组编辑潜力:
- 由于 TnpB 的 RNA 引导特性,科学家正在探索其作为基因组编辑工具的潜力。
- 它可能成为一种更小、更简单的替代方案,用于特定场景下的基因编辑。
- mRNA的自我加工
- TnpB能够加工自身的mRNA以产生引导RNA。这些引导RNA是短RNA分子,能够将TnpB核酸酶引导到特定的DNA序列。
- 加工过程涉及对mRNA的切割,生成引导RNA,随后引导RNA用于将核酸酶活性定位到基因组中的正确位置。
TnpB 与 CRISPR-Cas 系统的关系
- TnpB 被认为是 CRISPR-Cas 系统的进化前体之一。
- 它与 CRISPR-Cas 系统共享一些共同特征,例如 RNA 引导的 DNA 识别和切割机制。
- 研究 TnpB 有助于理解 CRISPR-Cas 系统的起源和进化。
研究意义
- 基础科学:
- 研究 TnpB 有助于揭示转座子的移动机制和 RNA 引导的核酸酶的功能。
- 它为理解 CRISPR-Cas 系统的进化提供了重要线索。
- 生物技术应用:
- TnpB 可能成为一种新型的基因组编辑工具,特别是在需要小型化或简化系统的场景中。
- 它还可以用于研究转座子的行为和基因组动态性。
转座酶类型
Transposases can be classified based on the mechanism of transposition, sequence specificity, and structural features.
1. DDE Transposases (RNase H-like transposases):
These transposases are named after a characteristic triad of acidic residues (Asp-Asp-Glu) in their catalytic domain. This family includes several well-studied transposases.
2. Tyrosine Transposases (Y1):
These typically use a tyrosine residue for transesterification reactions that facilitate transposition.
They often exhibit some similarity in mechanism to site-specific recombinases, which also use tyrosine for catalysis.
3. Serine Transposases:
These transposases utilize a serine residue in their catalytic mechanism.
Like other serine recombinases, they form a covalent protein-DNA intermediate during transposition.
4. HUH single-stranded DNA transposases (Rolling Circle (RC) Transposases, or Y2):
Also known as IS91-like transposases, these enzymes use a rolling-circle mechanism of transposition.
Unlike the cut-and-paste mechanism, RC transposition involves replication of the transposable element at the new site while the original remains in place.
5. Helitron Transposases:
Helitron transposons transpose by a rolling circle mechanism that is distinct from both classic DNA transposons and typical RC transposases. They are often studied in plants, where they play a significant role in genome evolution.
6. Transib Transposases:
This lesser-known class shares some features with both DDE and rolling-circle transposases but is distinguished by unique structural and sequence features. Transib transposases are found in a variety of organisms and are thought to have been involved in the evolution of the RAG recombinase crucial for vertebrate immune system function.
7. Reverse transcriptases/endonucleaseses (RT/En): combination of reverse transcriptase and endonuclease activities
Each class of transposases has evolved unique mechanisms and structural adaptations that allow them to facilitate the movement of their associated transposable elements. Their diversity reflects the wide range of roles they play in genomic rearrangement and evolution across different species.
DNA转座子类型
剪切-粘贴型DNA转座子/Cut-and-Paste transposon
CUT-AND-PASTE DNA TRANSPOSONS AS GENETIC TOOLS
Cut-and-paste DNA transposons are mobile genetic elements that can move from one genomic location to another through a process known as transposition. They “cut” themselves out of one location in the genome and “paste” themselves into a new location. This capability makes them an invaluable tool for genetic studies and biotechnological applications. Here, we explore the use of cut-and-paste DNA transposons as genetic tools.
Mechanism of Action
The classic cut-and-paste DNA transposons move through a “cut-and-paste” mechanism, which involves the following steps: 1. Recognition: The transposase enzyme, encoded by the transposon, binds to specific sequences at the ends of the transposon. 2. Excision: The enzyme cuts the DNA at these points, excising the transposon from its original location. 3. Integration: The transposase then mediates the integration of the transposon at a new site in the genome.
This process can occur without the need for DNA replication, which differs from the replication-based movement seen in some other types of transposable elements.
Applications as Genetic Tools
Cut-and-paste DNA transposons are used in a variety of ways in genetic research and biotechnology:
Gene Delivery: Transposons can be used to insert genes into cells, making them useful for gene therapy or creating transgenic organisms.
Mutagenesis: By inserting themselves into or near genes, transposons can disrupt gene function, helping researchers study gene roles and create model organisms with specific mutations.
Genome Manipulation: Transposons can be used to create insertions, deletions, and inversions in the genome, allowing for large-scale modifications and functional genomics studies.
Gene Tagging: Transposons can serve as molecular tags to label genes. This is particularly useful in forward genetics, where a phenotype is traced back to the responsible gene.
Transgenic Tool: They can be employed to stably integrate transgenes into the genome, which is essential in creating transgenic plants and animals for agriculture and research.
Functional Genomics: Transposons can be used for gene knockout libraries, enabling the systematic analysis of gene function.
Controlling Transposition
To harness transposons as tools, scientists have developed methods to control their activity: – Binary Systems: Use two components, one to express the transposase and another containing the transposable element, to control spatial and temporal transposition. – Inducible Promoters: These can regulate the expression of transposase, activating transposition only in the presence of specific inducers or under certain conditions.
Limitations and Challenges
While cut-and-paste DNA transposons are powerful tools, there are limitations and challenges to their use: – Random Insertion: Transposons can insert into random genomic locations, which might cause unintended mutations or disruptions. – Transposon Silencing: Over time, cells may silence transposons, limiting their utility for long-term expression studies. – Efficacy: The efficiency of transposase enzymes can vary, and not all transposons are active in all cell types or species.
In Silico Analysis and Modeling
To understand and predict the behavior of transposons, computational models and simulations can be used alongside experimental data. Programming tools and genetic algorithms can help in: – Analyzing transposon insertion patterns across genomes. – Predicting potential off-target effects and assessing risk factors. – Exploring evolutionary dynamics of transposable elements within populations.
Programming can be leveraged to automate these analyses, utilizing languages such as Python or R, combined with bioinformatics tools and databases.
In conclusion, cut-and-paste DNA transposons serve as versatile genetic tools with a wide range of applications in modern biology. However, their use requires careful management and understanding to fully leveraging their capabilities while minimizing unintended consequences. With ongoing advancements in genetic engineering and control mechanisms, the role of transposons as a genetic tool will likely continue to expand and evolve.
复制-粘贴型DNA转座子/Copy-out-paste-in transposon
译自:Copy-out–Paste-in Transposition of IS911: A Major Transposition Pathway
1.复制-粘贴型DNA转座子分类和分布
目前复制-粘贴型DNA转座子主要分布在原核生物中,已经发现多个IS家族属于这类转座机制,包括IS1,IS3(IS2、IS3,IS150),IS481,IS1595, IS21、IS30、IS256、IS110/IS1111、ISLre2、ISL3等。但根据本实验室研究发现在真核生物中也存在复制-粘贴型DNA转座子,但尚未见研究报道。
2. 复制-粘贴型DNA转座子结构(IS911)
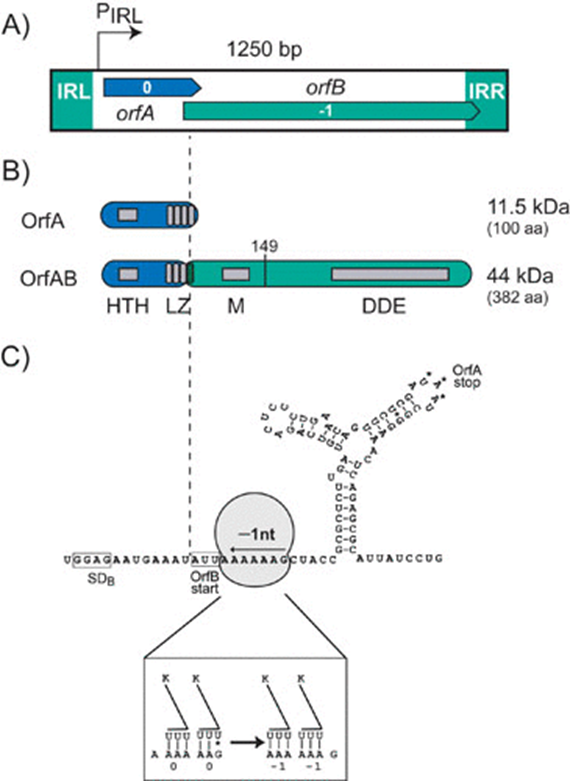
Organization of IS911. (A) Genetic organization. The 1,250-bp IS911 is shown as a box. The boxes at each end represent the left (IRL) and right (IRR) terminal inverted repeats. The two open reading frames, orfA (blue) and orfB (green) are positioned in relative reading phases 0 and −1, respectively, as indicated. The indigenous promoter, pIRL, is shown. The region of overlap between orfA and orfB, which includes the frameshifting signals to produce OrfAB, lies within IS911 coordinates 300 and 400. The precise point at which the frameshift occurs, within the last heptad of the LZ, is indicated by the vertical dotted line. (B) Structure function map of OrfAB and OrfA. HTH, a potential helix-turn-helix motif; LZ, a leucine zipper motif involved in homo- and hetero-multimerization of OrfAB and OrfA. Programmed translational frameshifting that fuses OrfA and OrfB to generate the transposase OrfAB occurs within the fourth heptad. The LZ of OrfA and OrfAB therefore differ in their fourth heptad. A second region, M, necessary for multimerization of OrfAB is shown, as is the catalytic core of the enzyme which carries a third multimerization domain. OrfA translation initiates at an AUG, terminates with UAA whereas OrfAB translation terminates within the right IR. The vertical line to the right of M shows the extent of the truncated transposase, OrfAB[1–149] described in the text. (C) Frameshifting window. The mRNA sequence around the programmed translational frameshifting window is presented. The boxed sequence GGAG is the potential ribosome-binding site located upstream of orfB whose potential translation would be initiated at the boxed AUU codon. A ribosome (not to scale) is shown covering a series of “slippery” codons (AAAAAAG). A downstream secondary structure is also shown with the UAA, OrfA translation termination codon. The ribosome-binding site, slippery codons and secondary structure all contribute to the efficiency of the programmed −1 frameshift. The box at the foot of this figure shows how the anti-codons of two tRNALys are thought to undergo re-pairing with their codons in the AAAAAAG motif.
3. 转座机制
以双链环状DNA为中介物,采用复制-粘贴机制进行转座,这个过程代表了一个重要的转座途径,但尚未得到广泛认识。简要来说,这个过程包括:末端配对;在一个IS末端进行切割,使得释放出的3’OH攻击另一个末端,形成一个由单链连接左右两端的“8”字形结构;随后复制生成一个两端相接的双链IS DNA圆环,在此同时,再生原始的供体分子。然后,转座酶断开双链的两个末端,并将它们连接到靶点上(图5)。
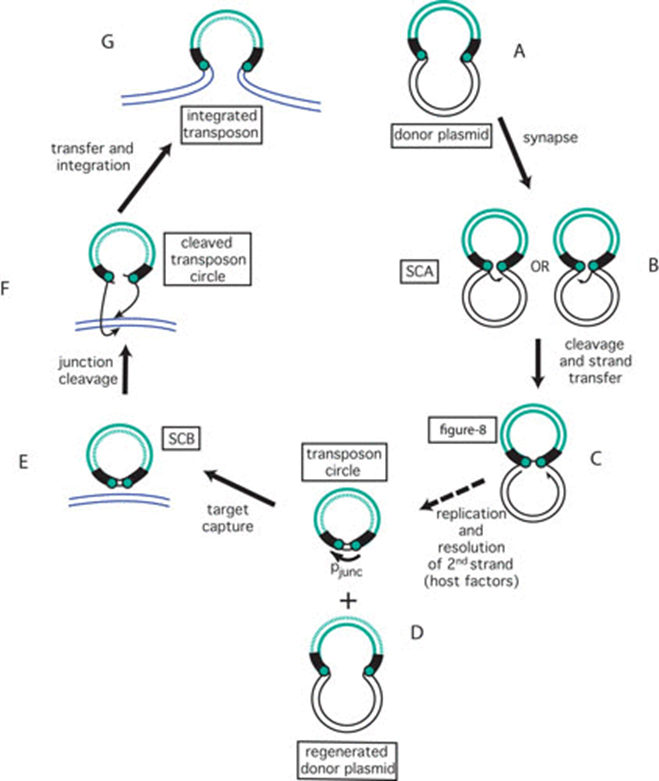
图5. IS911转座循环。
转座子以绿色显示,侧翼供体DNA为黑色,靶DNA为蓝色。转座子末端显示为绿色实心圆。图4中显示的小箭头因简略而省略。(A)携带插入序列(IS)的供体质粒。(B)形成首个突触复合物SCA,切割左侧或右侧的倒置重复序列(IR),并攻击另一端。(C)供体质粒形成单链桥以创建“8”字形分子。(D)IS特异性复制的产物:双链圆形IS转座中间体和再生的转座子供体质粒。复制的链以绿色虚线显示。(E)形成第二个突触复合物SCB并接合靶DNA(蓝色)。(F)切割IS圈并整合。(G)新整合的IS。(The IS911 transposition cycle. The transposon is shown in green, the flanking donor DNA in black and the target DNA in blue. Transposon ends are shown as green filled circles. The small arrows shown in Figure 4 have been omitted for brevity. (A) Donor plasmid carrying the insertion sequence (IS). (B) Formation of the first synaptic complex SCA and cleavage of the left or right inverted repeat (IR) and attack of the other end. (C) Formation of a single-strand bridge to create a figure-eight molecule if the donor is a plasmid as shown here. (D) The products of IS-specific replication: the double strand circular IS transposition intermediate and the regenerated transposon donor plasmid. The replicated strand is shown as a green dotted line. (E) Formation of the second synaptic complex SCB and engagement of the target DNA (blue). (F) Cleavage of the IS circle and integration. (G) The newly integrated IS.)
4. “8”字形分子的形成
区别于其他转位机制的关键特征在于单侧IS末端进行不对称切割并在5’末端自我连接。这样,通过单链桥将两个末端连接起来,在圆形质粒供体分子上生成一个“8”字形中间体。 该途径的初始步骤是OrfAB识别IR(这可能发生在其翻译期间)并组装SCA(见上文),以正确定位DNA末端和转座酶的催化位点,进行随后的化学步骤(图5A和B)。 和所有已知的DDE转座酶催化反应一样,IS911的转位通过单链在转座子末端的切割生成一个3’OH开始。这个3’OH随后攻击目标磷酸二酯键进行链转移反应。这种复制-粘贴机制的特点是,初始切割只发生在转座子的一个末端,无论是左边还是右边(图5B)。 这个单一释放出的3’OH指导链转移至同一个链,在另一端的5’方向上三个碱基。这生成了一种分子状态,在圆形质粒供体分子上,一个单一的转座子链被环化,产生单链桥,形成“8”字结构(图5C),这可以在体内轻易观察到(73)。 这两个IR通过单链桥连接,并与左或右端的侧翼DNA衍生的三个碱基隔开。原始插入位点两侧的3(或4)个碱基直接重复序列对于转位进程并非必需(IS3也有同样的情冁,见74),一个基于IS911的转座子被设计为具有不同的侧翼片段,生成一个带有一侧或另一侧侧翼序列的混合“8”字形分子群体。防止切割转座子的任一端,会产生一个均质群体,它携带与突变端相关的三核苷酸DNA侧翼,证实了IRL可以攻击IRR,反之亦然。这个反应可以被视为单端位点特异性的转位事件。此类单端攻击作为IS2的正常途径发生。这个IS在一个末端携带一个突变,阻止它被切割并作为攻击性供体链。 这些初始步骤可以由OrfAB独立完成。但需要注意的是,在OrfA存在的情况下,虽然通过PCR方法可以发现IS圈(46),但通过体内简单的凝胶检测却无法检测到“8”字形或IS圈。这表明OrfA在负向调控转位启动方面可能起作用。对于IS3的OrfA也得出了类似的结论(75)。或者,OrfA可能刺激“8”字形和IS圈的消失(见下文),因为在体外OrfA对“8”字形的形成没有观察到影响(76)。加上OrfAB通常由一个弱启动子产生少量表达的事实(15),形成“8”字形中间体的转位启动可能是随机的。
5. 环状中间体的形成
通过复制,从“8”字形中间体产生一个双链圆形IS中间体。动力学数据(37,73)已经证实,“8”字形能够生成圆形转座子形式,该形式能够在体内轻易检测到(77)。在IS圈中的IR被拼接在一起,原始插入点两侧的3个DNA碱基将其分开(图5D)。和“8”字形分子一样,经过工程设计具有不同侧翼片段的转座子能够产生转座子环混合体,其中一个或另一个3碱基侧翼位于连接点(77)。
进一步的体内研究(采用分阶段标记方法结合温敏性质粒作为转座子供体)证明了从“8”字形到转座子环的转变是通过半保留复制进行的,其中圆形中间体被“复制出来”,留下一个副本在转座子供体分子中(图5D)(78)。这是转座子特异性的,需要OrfAB(推测是为了生成“8”字形,并在IS911 DNA侧翼产生一个3’OH)而且这个过程不依赖于供体质粒的复制起点(78)。
使用其中一个IR被停用的供体质粒可以预期地决定哪一个3’OH被用于转座子复制。通过Tus/ter系统(79-82)进行测试(该系统能够以方向特异性的方式阻止复制叉的通过),将其克隆到转座子的一个或另一个方向中。在Tus蛋白存在的情况下,如果ter位点的方向预计会阻止从一个或另一个末端开始复制,那么就观察不到转座子环(78)。这证明复制是使用供体侧翼的3’OH进行的。因此,环形成可以被描述为一种以供体为引发的转座子复制机制。
目前,尚不清楚OrfAB如何被移除,以及如何启动或终止这一复制步骤来生成最终的环。这些过程涉及宿主因子和机制有可能类似于噬菌体Mu复制性转位所操作的(参见本卷的Harshey)(83, 84)。
6. 圆形中间体的整合
圆形IS中间体(insertion sequence, IS)被切割以释放3’羟基,该羟基随后攻击目标DNA,完成最终的整合步骤。事实上,研究也表明,在OrfAB存在的情况下,IS环状化形成的IR结(inverted repeat junction)非常不稳定,在体内(85)和体外(70)会发生高水平的缺失和插入。 据推测,转座子环的插入需要进一步合成转座酶。转座子环形成的一个显著后果是,一个强大的启动子pjunc由IRR贡献的-35六聚体和IRL贡献的-10六聚体组装而成(见图2B;图5D)。在环中分隔IRL和IRR的3个(或较少地4个)碱基为-35和-10元素提供了理想的间距(85)。交接启动子pjunc比内源启动子pIRL强30-50倍(85),也比lacUV5强两倍以上(14)。它放置得当,能够驱动高水平的转座酶合成,并在控制IS911转座中发挥积极作用。通过诱变学灭活pjunc,当转座酶以其原生构型表达时,显著减少了体内的IS911转座(14)。此外,缺陷的OrfAB衍生物OrfAB[1-149],它特异性结合IRR和IRL,在一种抗切割的突变交接处将体内启动子活性降低了10倍。全长的OrfAB,它仅微弱地结合IR,以及OrfA,它不特异性结合IR,都没有影响(14)。在与IS2相关的情况下,这个交接启动子对转座是必需的(86)。整合结果导致pjunc解体,提供了一个强大的反馈机制,导致在正确(圆形)中间体存在时瞬时和控制的整合激活。 圆形结合位点的形成将两个转座子末端以倒置的方向汇集在一起。然后这个活跃的结合位点必须参与到包含目标DNA的第二种类型的突触复合体中(见图5E)。插入可以遵循两条路径:非靶向或靶向。 在非靶向路径中,插入相对序列独立。两个单链切割,每个紧邻的IR一个,线性化转座子环,允许两个释放的3’羟基指导协同的链转移(见图5F)。最后一步需要OrfAB,但极大地由OrfA刺激,并且对OrfAB/OrfA的比例敏感(70)。尚不清楚靶向捕获是在圆形结合位点切割之前还是之后发生,尽管观察到在体外和体内OrfAB水平高时,从转座子环生成IS911的线性副本,而且体外预先切割的线性转座子是一种强有力的整合底物(87)。基于动力学和强大的pjunc启动子的形成,我们更倾向于一个模型,在此模型中,IS环表示转座中间体的一个储备,并且在整合过程中从IS环生成线性形式。这同样也被提出用于IS3(74)。对于相关的IS150,也已经鉴定了IS环和线性IS形式(3, 74, 88)。 在靶向路径中,一个突触复合体在转座子环的IR和目标中的IR样序列之间形成。看起来只有单一的IS末端被切割并转移到“目标”位点。这个过程涉及目标IS911末端,链转移发生在IS环的一个切割末端和目标IS末端之间,创建了一个分子间的单链桥,而不是图中八字形中间体的分子内桥(见图4B左侧)。这一结构的解析涉及分支迁移和来自供体质粒的复制(89)。在这方面,RecG解旋酶在这个靶向插入过程中被暗示有作用。
Reference:
Copy-out–Paste-in Transposition of IS911: A Major Transposition Pathway
科研成果
课题组Mohamed博士在《Advanced Science》期刊(IF:15.1,国际顶级期刊,1区)发表Spy转座子研究论文,2024.4
课题组石莎莎博士在国际学术期刊Molecular Phylogenetics and Evolution (IF:4.1,1区)上发表Hiker转座子研究论文,2023.8
PS和ZB转座子系统及配套专利技术成果转让上海细胞治疗集团有限公司,2023.4
课题组王赛赛博士后在国际学术期刊Nucleic Acids Research (IF:14.90,2区)上发表PS转座子研究成果,2023
课题组沈丹博士在国际学术期刊Nucleic Acids Research (IF:19.16,1区)上发表ZB转座子研究成果,2021
基因治疗——微信平台
转座子科普——微信平台
Cas9基因编辑效率提升百倍,打造基因编辑通用型改造策略,助力治疗基因疾病,2024-7
两篇Nature Chemical Biology|小尺寸大作为:IscB在基因技术领域的潜力爆发,2024-7
转座酶辅助下的高效植物基因组整合技术—TATSI-2024-7
转座子的研究现状:王露课题组-2024-7
IscB在基因技术领域的潜力爆发-2024-7
植物基因组高效无缝插入技术正式问世-2024-6
seekRNA为精确的基因编辑提供了新的技术,2024-6
新基因编辑工具SeekRNA面世-2024-6
seekRNA为精确的基因编辑提供一种新途径-2024-6
桥RNA介导的大片段基因高效精准定点整合技术-2024-7
桥RNA介导的大片段基因高效精准定点整合技术-2024-7
双特异性桥RNA介导大片段DNA的精准重排-2024-7
Nature | 转座子编码的核酸酶利用向导RNA促进其自身扩散
Science | 张峰组解析出非长末端重复逆转录转座子起始逆转录的结构基础
在基因组中“跳来跳去”的转座子,有望成为免疫疗法抗击肿瘤的新靶点!
Life Med亮点 | 逆转座子调控人类早期胚胎发育及疾病发生
Nature Reviews Genetics | 转座子元件对哺乳动物染色质结构的作用
相册
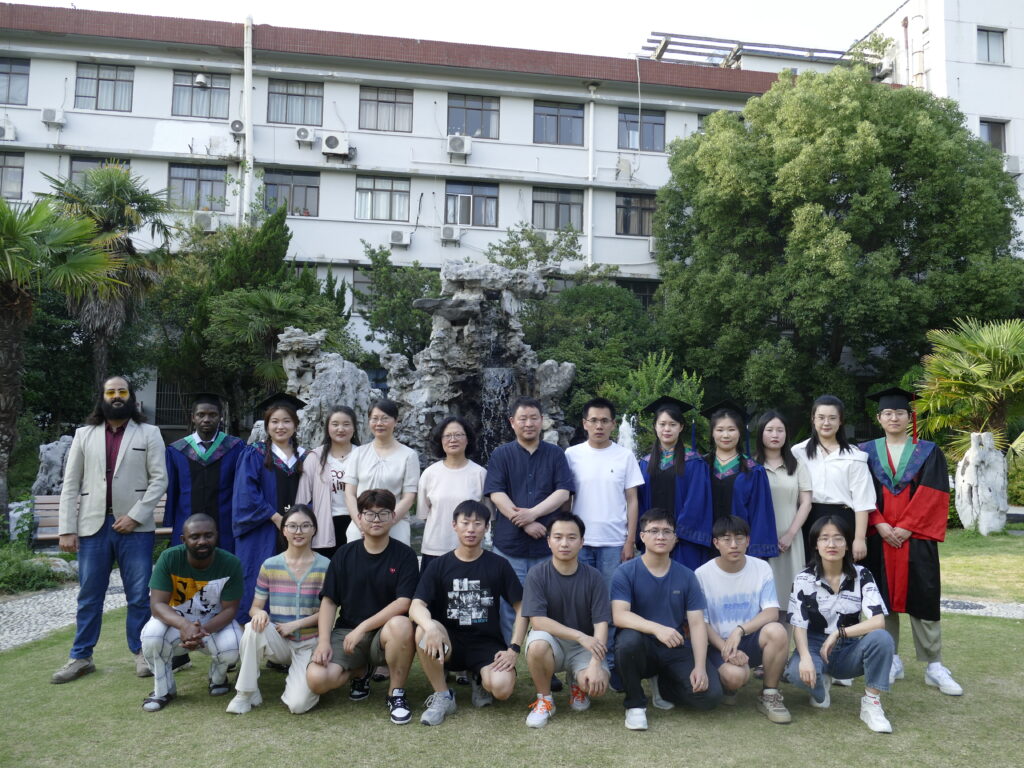
2024-6 王赛赛(博士后)、杨乃苏(博士)、硕士郭梦可、何佳、袁文娟、ADDY合影
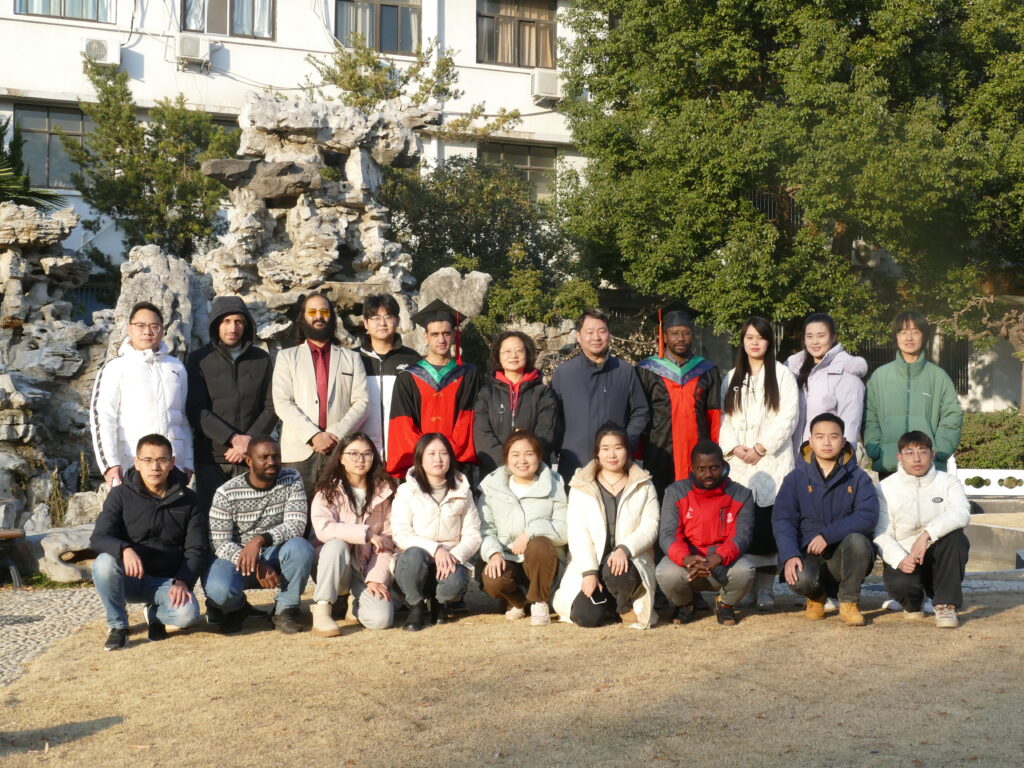
2023-12-Numan and Mohamed Ph.D graduation
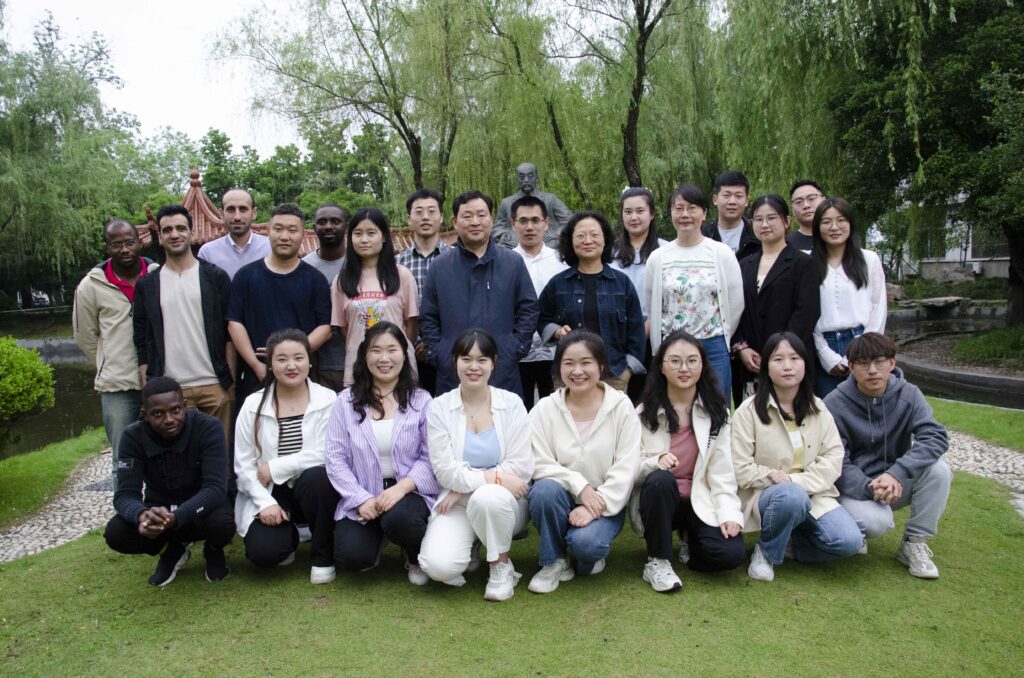
2023-6 王亚丽(博士)、杜站宇(博士)、贾文竹、王梦礼毕业合影
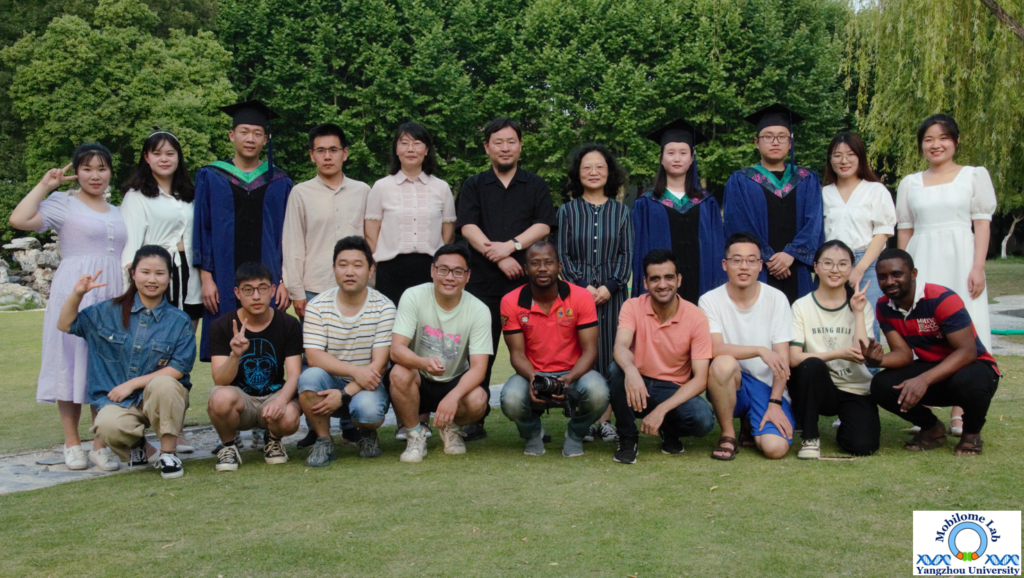
2022-6 关中夏、石莎莎、迟城林毕业合影
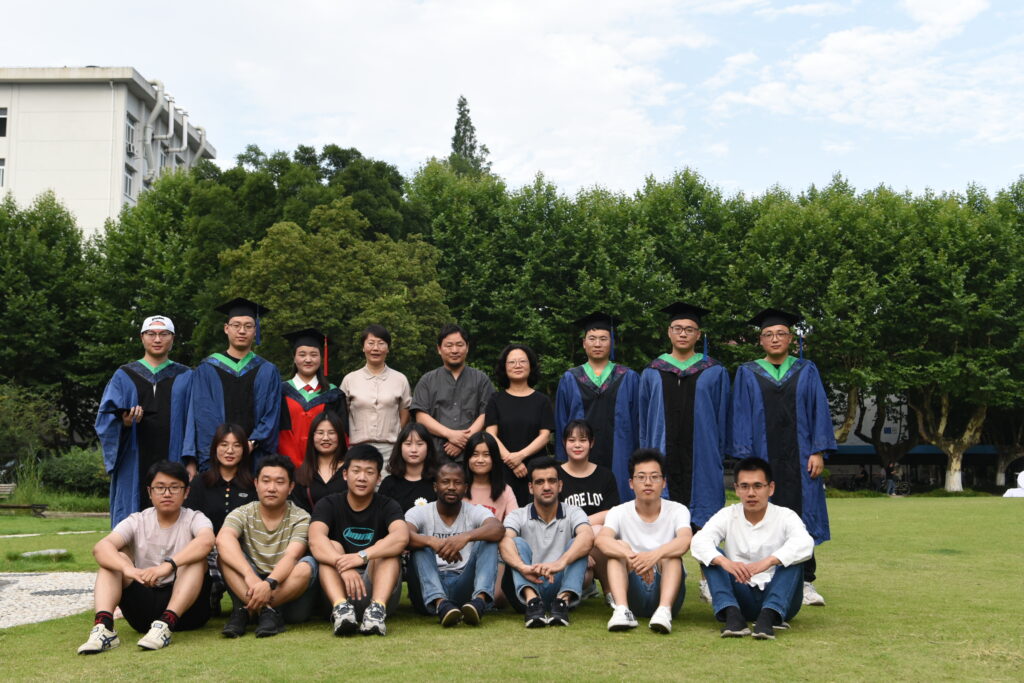
2021-王赛赛(博士)、宗文成、郑尧、桑亚通、安亚龙、顾浩毕业合影
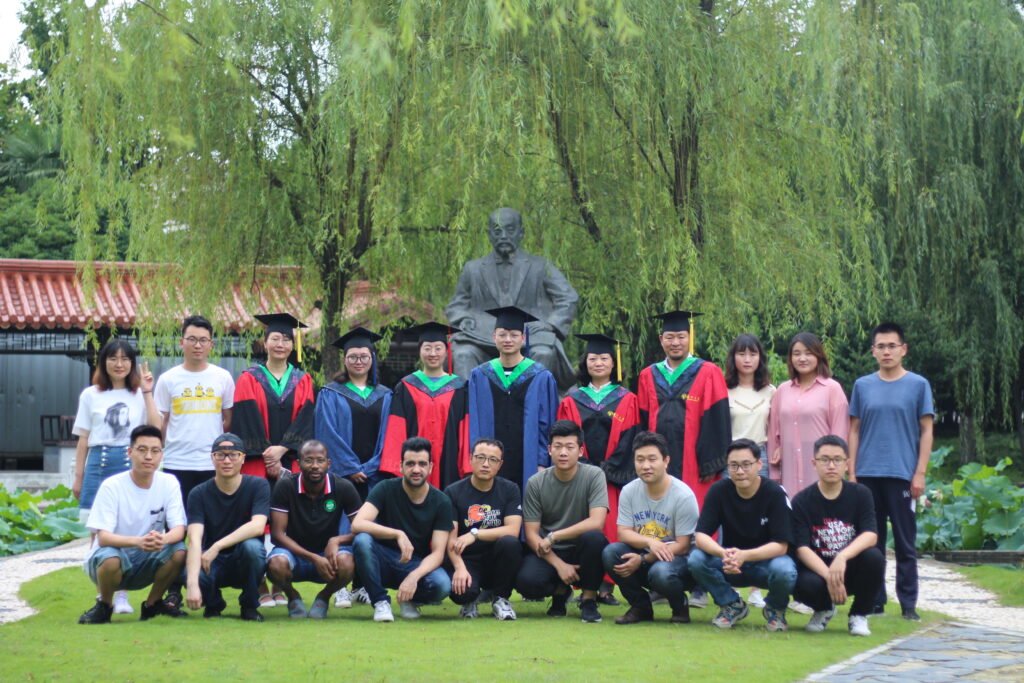
2020-沈丹、产舒恒、陈子旋毕业合影
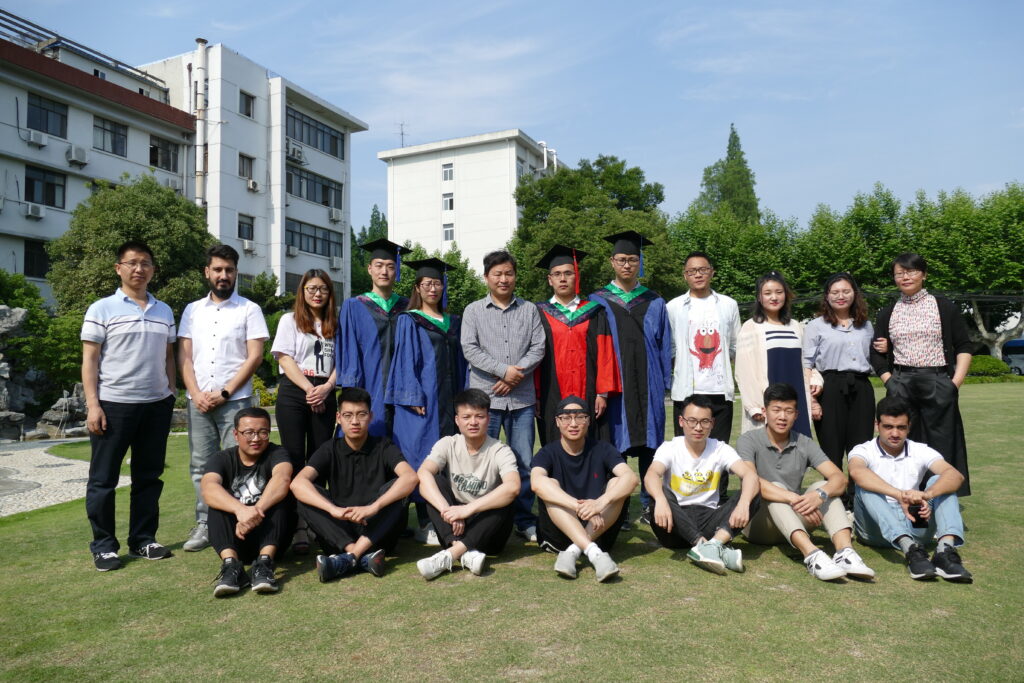
2019-6 陈才(博士)、王伟、陈伟、张丽毕业合影
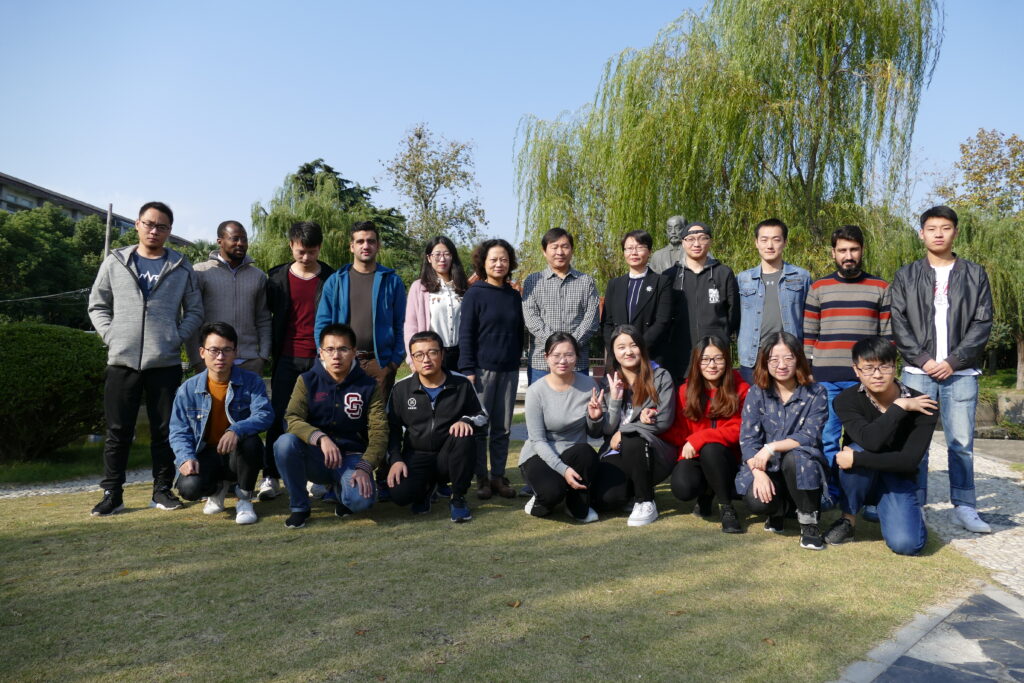
2018-12 张丽毕业合影
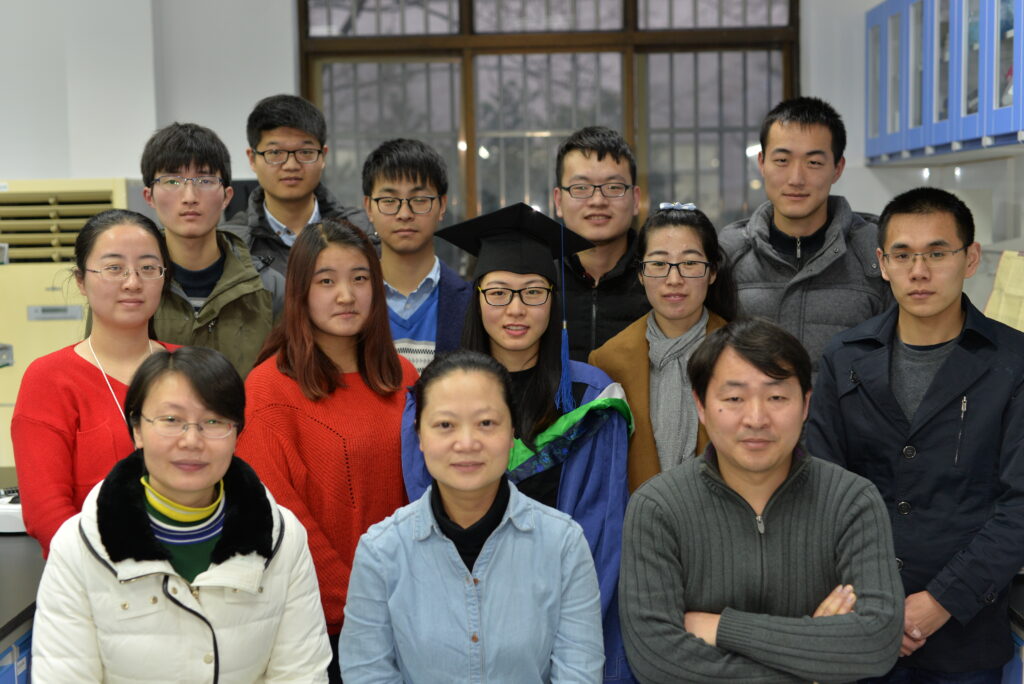
2017-12 王亚丽、刘帅军、钟继汉毕业合影
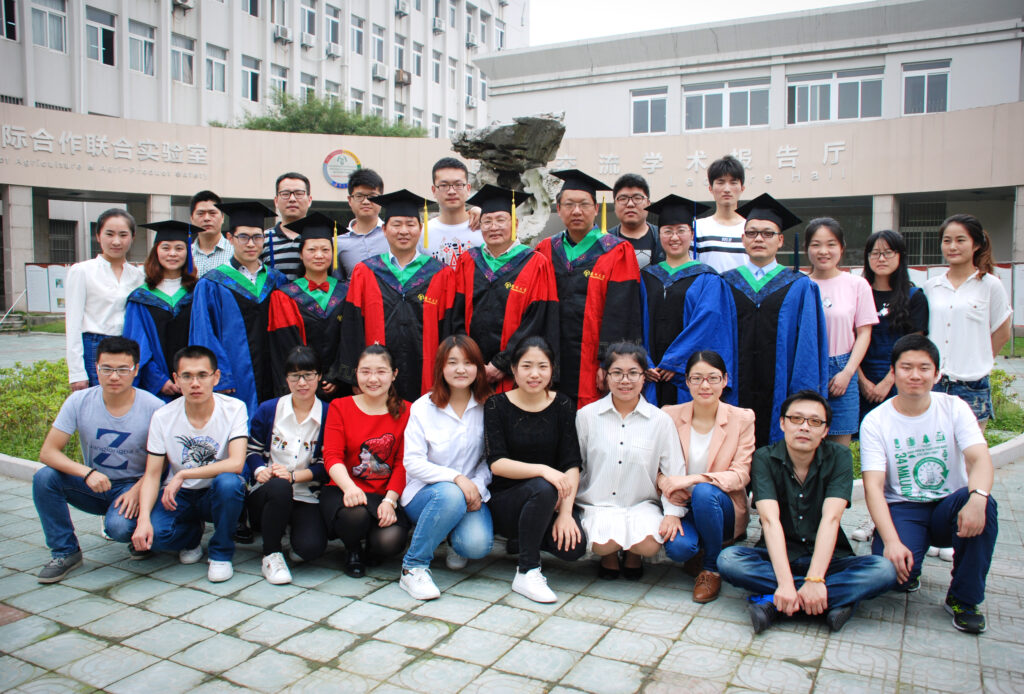
2016-6 沈丹、薛松磊毕业合影
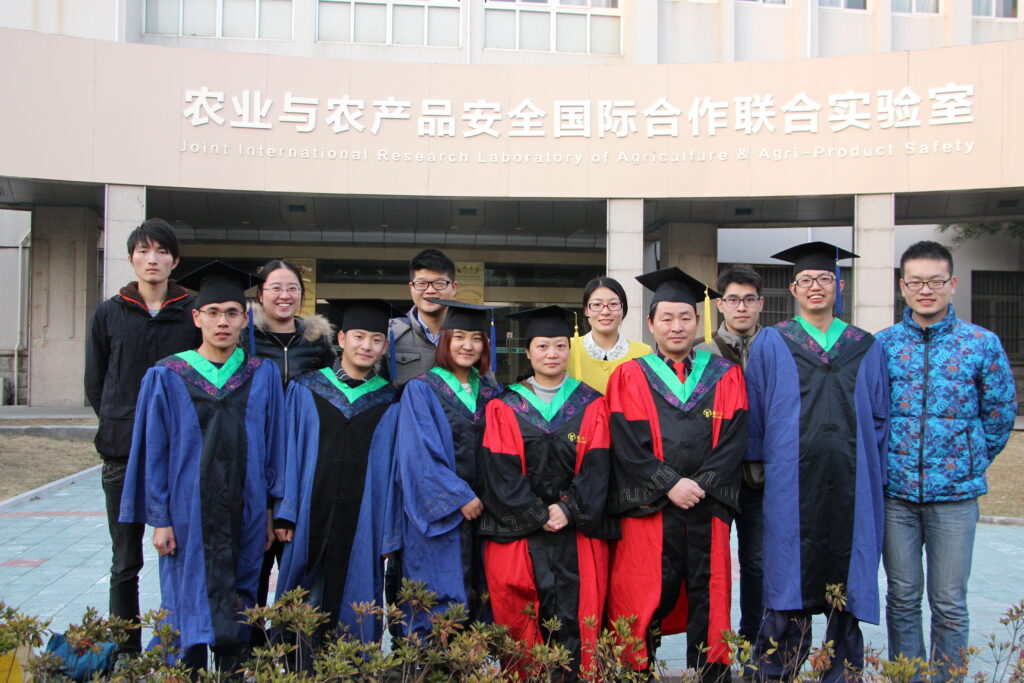
2015-12 王赛赛、陈才、钱跃、史云强
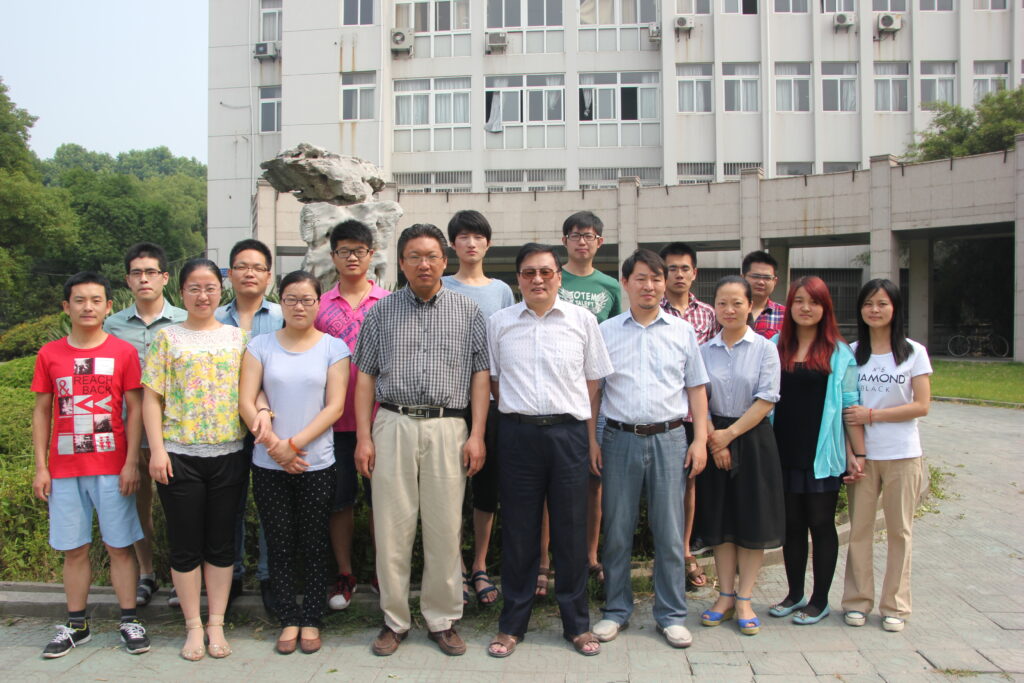
2014 YearBook
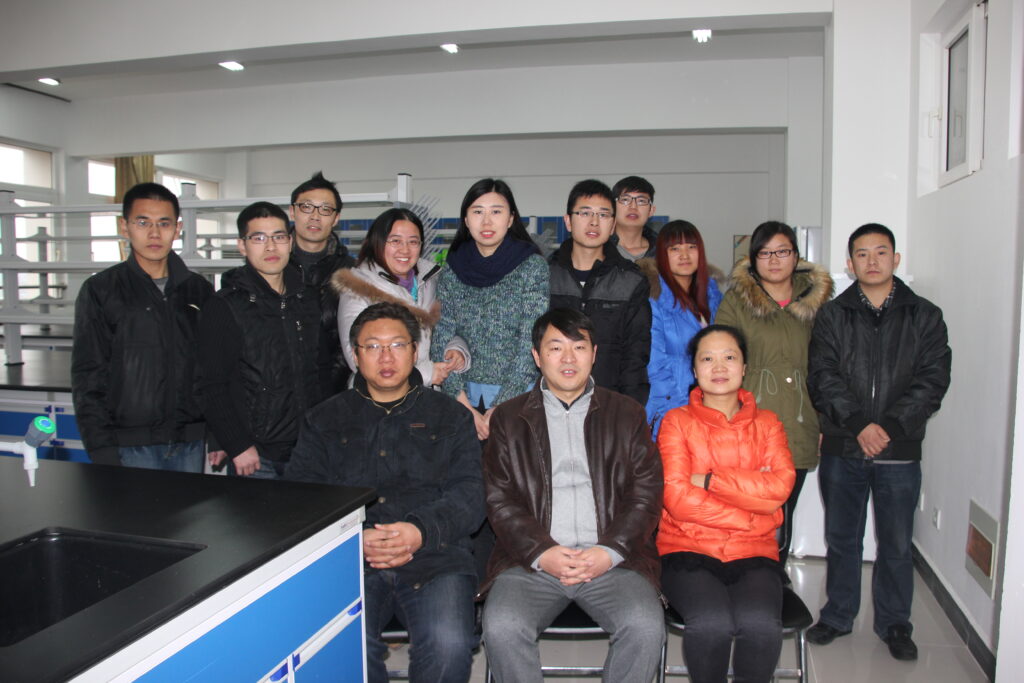
2013 YearBook
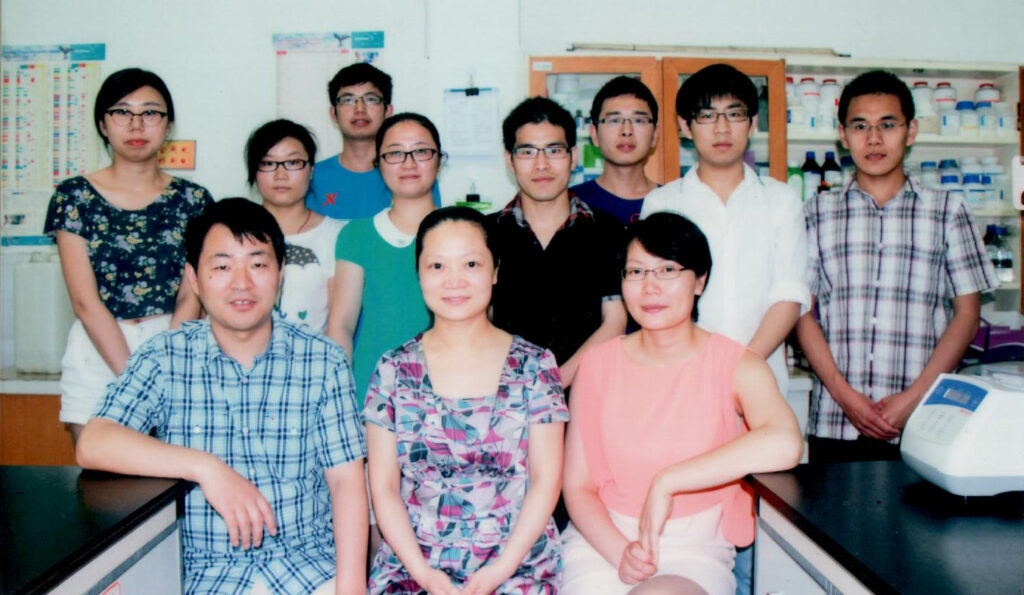
2013 YearBook
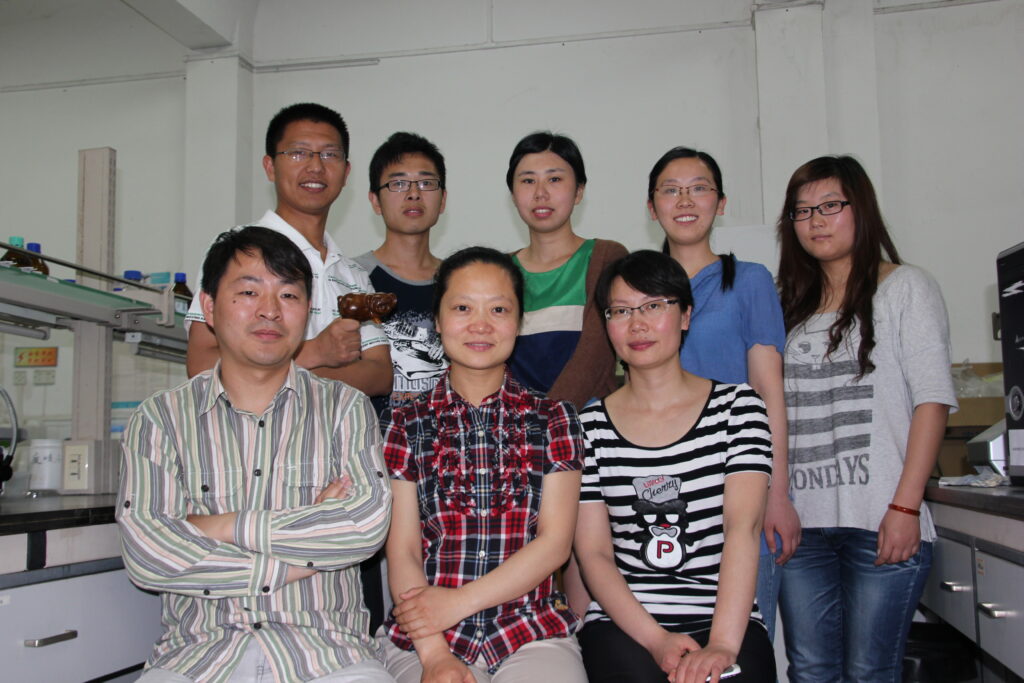
2012 YearBook
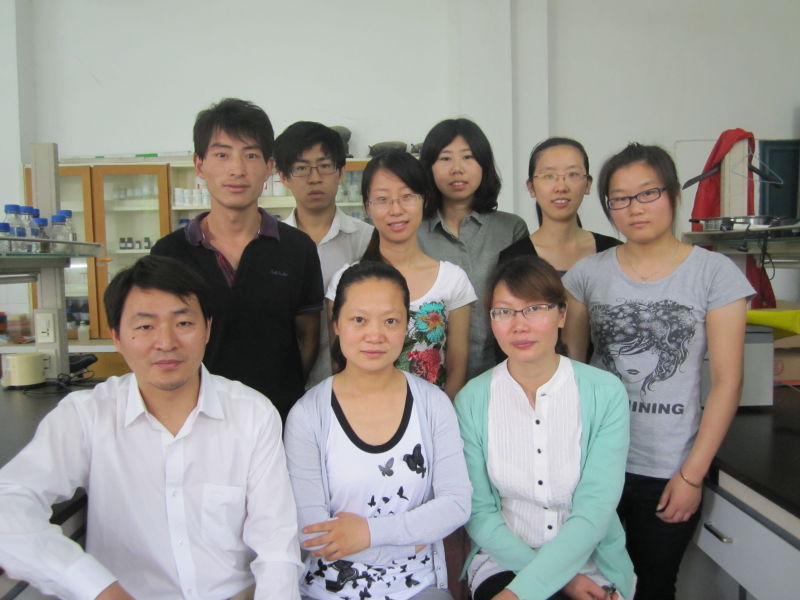
2011 YearBook
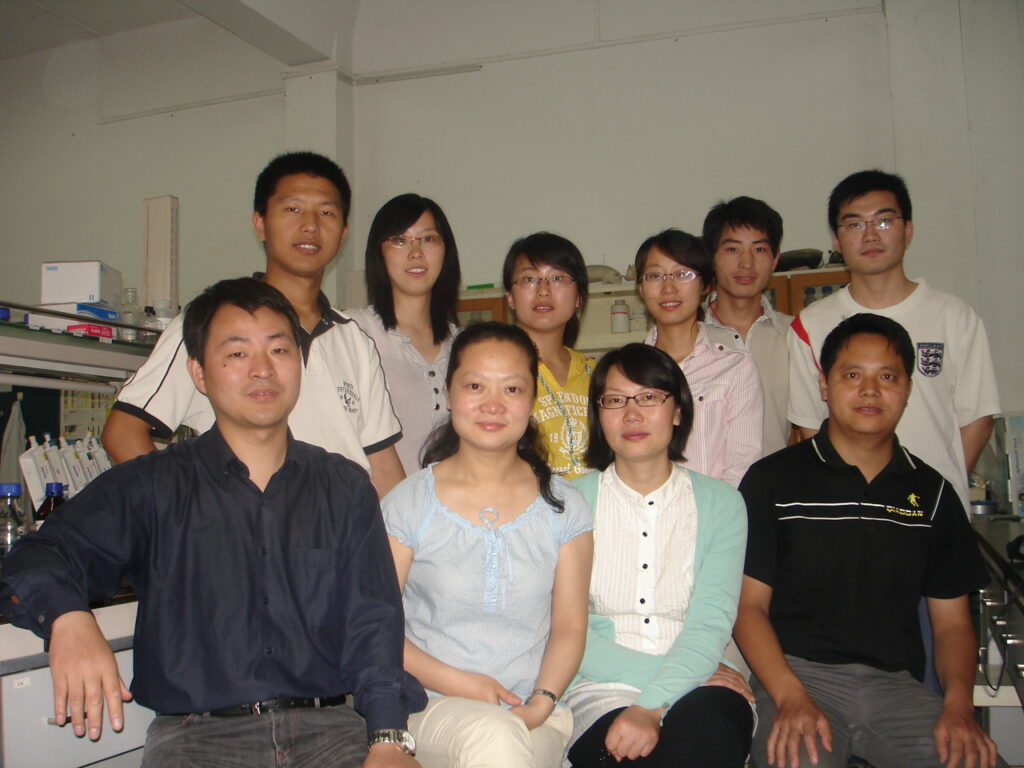
2010 YearBook-1
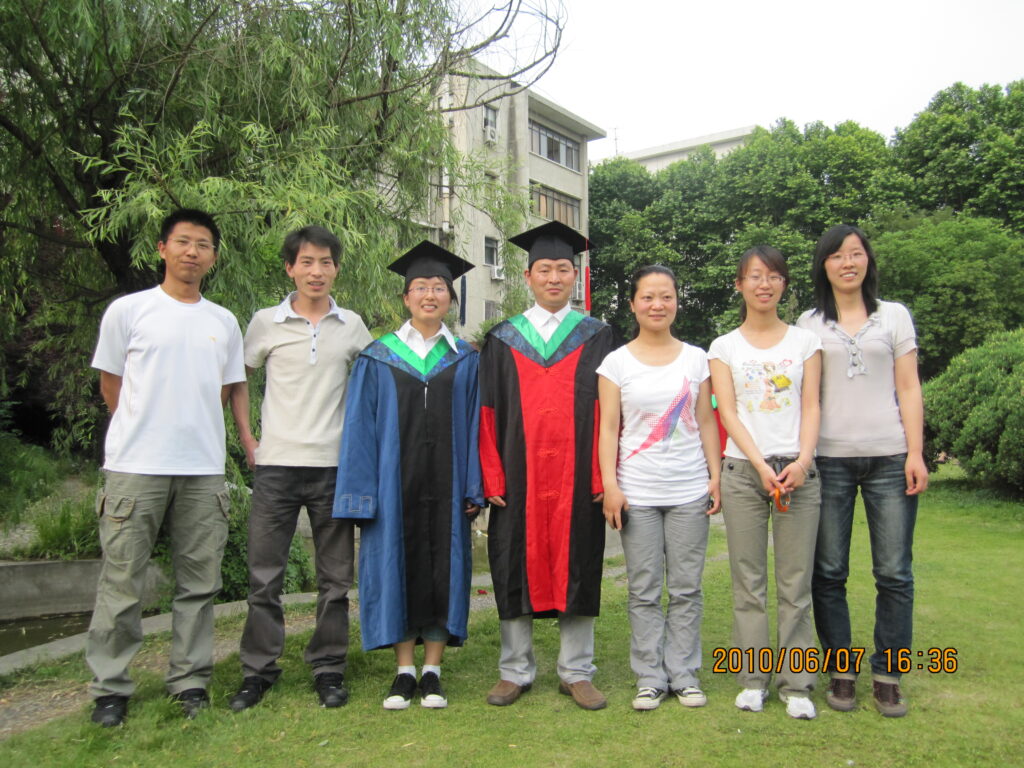
2010 YearBook-2
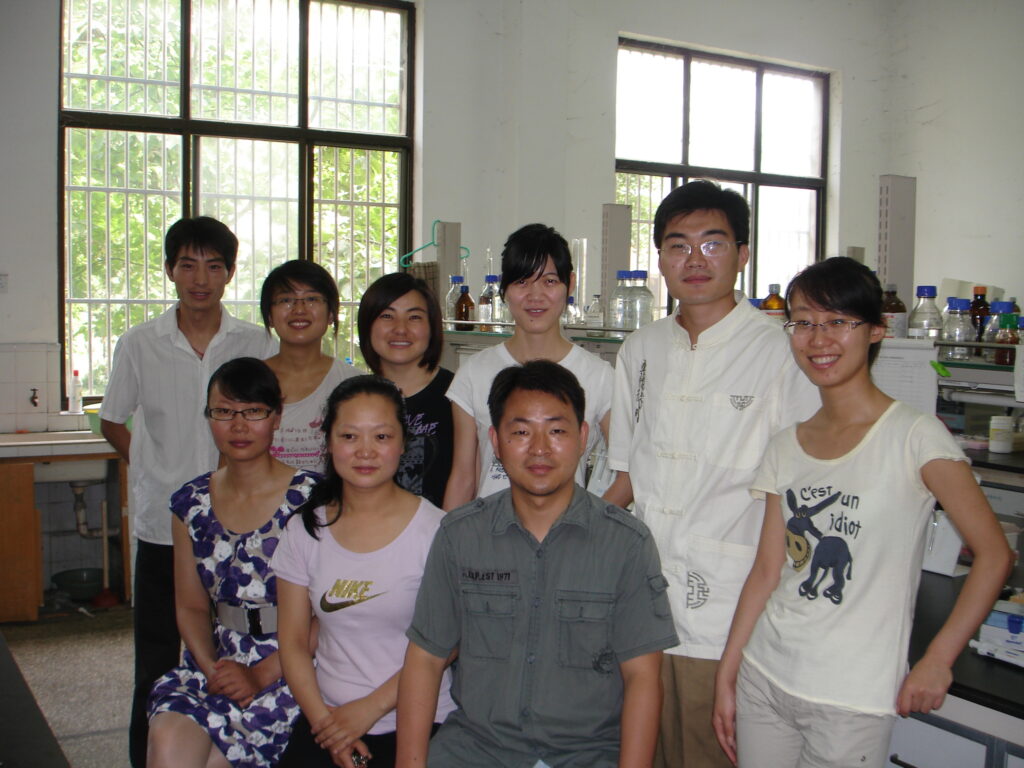
2009 YearBook
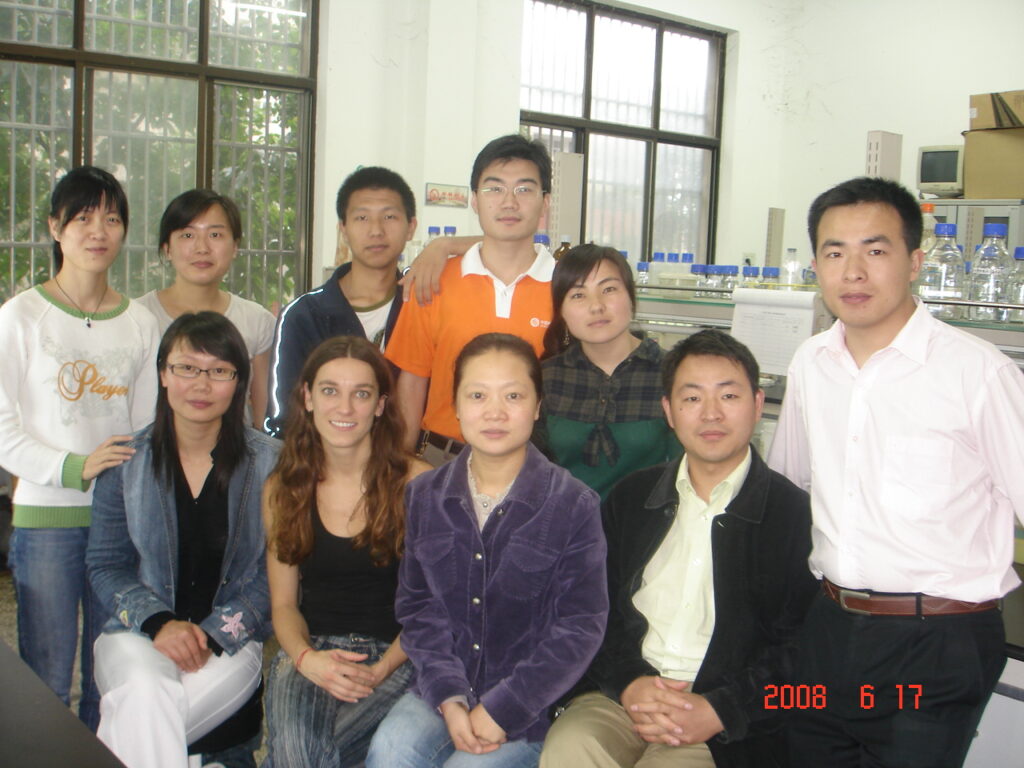
2008 YearBook-1
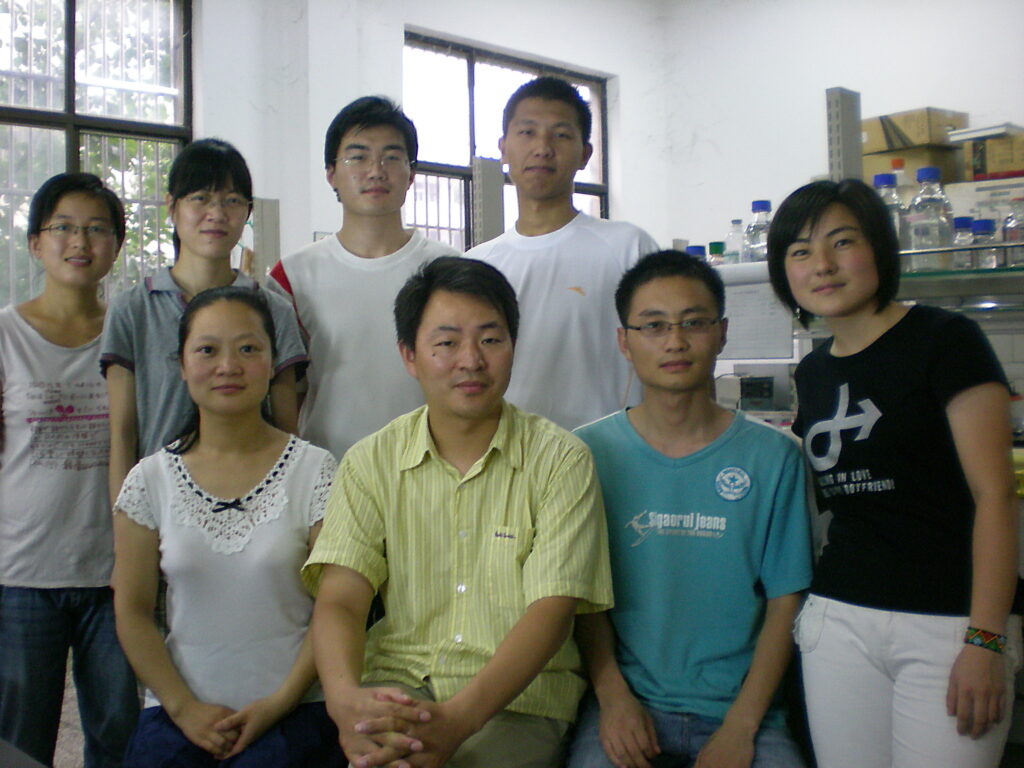
2008 YearBook-2
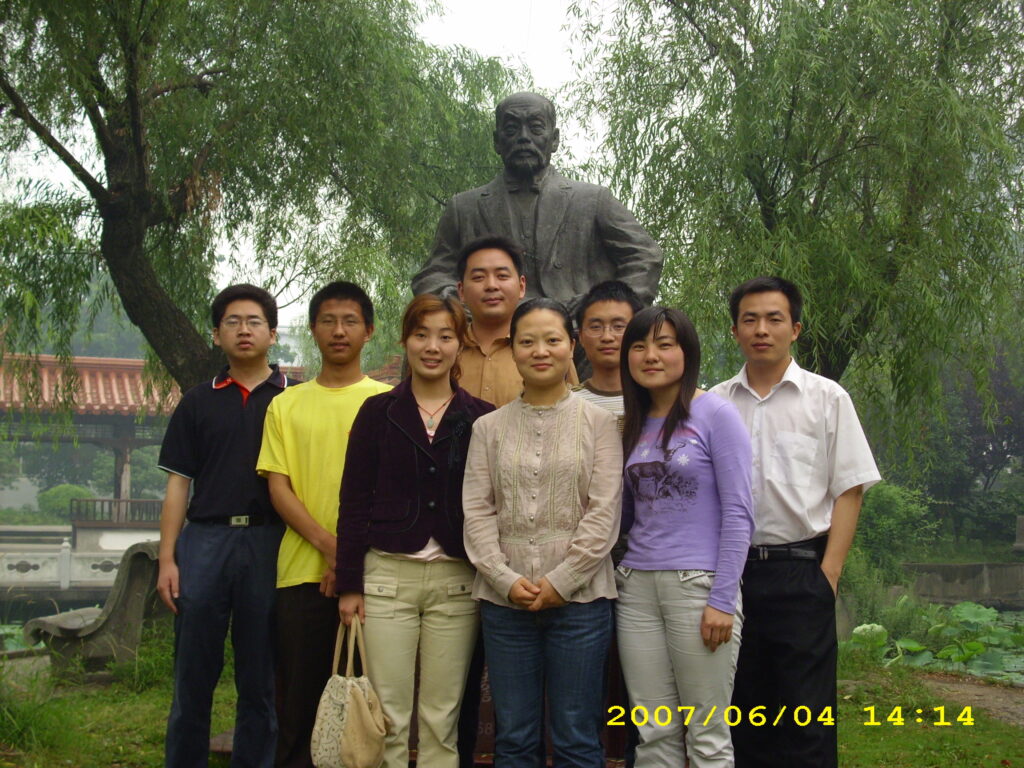
2007 YearBook
Key TE Reviews
Transposable elements, contributors in the evolution of organisms (from an arms race to a source of raw materials), Heliyon. 2021. PMID: 33532648.
Specificities and Dynamics of Transposable Elements in Land Plants. Biology (Basel). 2022. PMID: 35453688
A Field Guide to Eukaryotic Transposable Elements. Annu Rev Genet. 2020. PMID: 32955944.
A survey of transposable element classification systems–a call for a fundamental update to meet the challenge of their diversity and complexity. Mol Phylogenet Evol. 2015. PMID: 25797922.
All Quiet on the TE Front? The Role of Chromatin in Transposable Element Silencing. Cells. 2022. PMID: 36010577.
An epigenetic toolkit allows for diverse genome architectures in eukaryotes. Curr Opin Genet Dev. 2015. PMID: 26649755.
Bridging multiple dimensions: roles of transposable elements in higher-order genome regulation. Curr Opin Genet Dev. 2023. PMID: 37028152.
Coevolution between transposable elements and recombination. Philos Trans R Soc Lond B Biol Sci. 2017. PMID: 29109221.
Constitutive Heterochromatin in Eukaryotic Genomes: A Mine of Transposable Elements. Cells. 2022. PMID: 35269383.
Dark genome, bright ideas: Recent approaches to harness transposable elements in immunotherapies. Cancer Cell. 2022. PMID: 35907399.
Distinguishing friends, foes, and freeloaders in giant genomes. Curr Opin Genet Dev. 2018. PMID: 29544119.
Diversity and evolution of transposable elements in Arabidopsis. Chromosome Res. 2014. PMID: 4801342.
Double-edged sword: The evolutionary consequences of the epigenetic silencing of transposable elements. PLoS Genet. 2020. PMID: 32673310.
Epigenetic regulation of intragenic transposable elements: a two-edged sword. J Biochem. 2018. PMID: 30010918.
Evolution of transposable elements and evolution of eukaryote genomes mediated by transposable elements. Genes Genet Syst. 2019. PMID: 31996493.
Evolutionary impact of transposable elements on genomic diversity and lineage-specific innovation in vertebrates. Chromosome Res. 2015. PMID: 26395902.
Giant Transposons in Eukaryotes: Is Bigger Better? Genome Biol Evol. 2019. PMID: 30796812.
Horizontal acquisition of transposable elements and viral sequences: patterns and consequences. Curr Opin Genet Dev. 2018. PMID: 29505963.
Horizontal transfers of transposable elements in eukaryotes: The flying genes. C R Biol. 2016. PMID: 27234293.
Host Gene Regulation by Transposable Elements: The New, the Old and the Ugly. Viruses. 2020. PMID: 32993145.
Host-transposon interactions: conflict, cooperation, and cooption. Genes Dev. 2019. PMID: 31481535.
How retrotransposons shape genome regulation. Curr Opin Genet Dev. 2016.PMID: 26855260.
Impact of transposable elements on the evolution of complex living systems and their epigenetic control. Biosystems. 2021. PMID: 34718084.
Integration of TE Induces Cancer Specific Alternative Splicing Events. Int J Mol Sci. 2022. PMID: 36142830.
Integration site selection by retroviruses and transposable elements in eukaryotes. Nat Rev Genet. 2017. PMID: 28286338.
Investigating the Potential Roles of SINEs in the Human Genome. Annu Rev Genomics Hum Genet. 2021. PMID: 33792357.
Jumping the fine LINE between species: horizontal transfer of transposable elements in animals catalyses genome evolution. Bioessays. 2013. PMID: 24003001.
Mammalian genome evolution as a result of epigenetic regulation of transposable elements. Biomol Concepts. 2014. PMID: 25372752.
Mammalian genome innovation through transposon domestication. Nat Cell Biol. 2022. PMID: 36008480.
Mammalian transposable elements and their impacts on genome evolution. Chromosome Res. 2018. PMID: 29392473.
Massive contribution of transposable elements to mammalian regulatory sequences. Semin Cell Dev Biol. 2016. PMID: 27174439.
Methodologies for the De novo Discovery of Transposable Element Families. Genes (Basel). 2022. PMID: 35456515.
Mobility connects: transposable elements wire new transcriptional networks by transferring transcription factor binding motifs. Biochem Soc Trans. 2020. PMID: 32573687.
More than causing (epi)genomic instability: emerging physiological implications of transposable element modulation. J Biomed Sci. 2021. PMID: 34364371.
New Insights on the Evolution of Genome Content: Population Dynamics of Transposable Elements in Flies and Humans. Methods Mol Biol. 2019. PMID: 31278675.
On the Importance to Acknowledge Transposable Elements in Epigenomic Analyses. Genes (Basel). 2019. PMID: 30935103
Recent Bioinformatic Progress to Identify Epigenetic Changes Associated to Transposable Elements. Front Genet. 2022. PMID: 35646069.
Regulatory activities of transposable elements: from conflicts to benefits. Nat Rev Genet. 2017. PMID: 27867194
Repetitive elements in aging and neurodegeneration. Trends Genet. 2023. PMID: 36935218.
Retrotransposons as a Source of DNA Damage in Neurodegeneration. Front Aging Neurosci. 2022. PMID: 35058771.
Rewiring of chromatin state and gene expression by transposable elements. Dev Growth Differ. 2021. PMID: 34050925.
Role of Transposable Elements in Gene Regulation in the Human Genome. Life (Basel). 2021. PMID: 33557056.
Role of Transposable Elements in Genome Stability: Implications for Health and Disease. Int J Mol Sci. 2022. PMID: 35887150.
Roles of Transposable Elements in the Different Layers of Gene Expression Regulation. Int J Mol Sci. 2019. PMID: 31731828.
Roles of transposable elements in the regulation of mammalian transcription. Nat Rev Mol Cell Biol. 2022. PMID: 35228718.
Silencing of Transposable Elements by piRNAs in Drosophila: An Evolutionary Perspective. Genomics Proteomics Bioinformatics. 2017. PMID:28602845.
Structural and sequence diversity of eukaryotic transposable elements. Genes Genet Syst. 2020. PMID: 30416149.
Taming the Turmoil Within: New Insights on the Containment of Transposable Elements. Trends Genet. 2020. PMID: 32473745.
Taming transposable elements in vertebrates: from epigenetic silencing to domestication. Trends Genet. 2022. PMID: 35307201.
Taming, Domestication and Exaptation: Trajectories of Transposable Elements in Genomes. Cells. 2021. PMID: 34944100.
TFs for TEs: the transcription factor repertoire of mammalian transposable elements. Genes Dev. 2021. PMID: 33397727.
The challenges of predicting transposable element activity in hybrids. Curr Genet. 2021. PMID: 33738571.
The evolution and function of transposons in epigenetic regulation in response to the environment. Curr Opin Plant Biol. 2022. PMID: 35961279.
The Evolutionary Volte-Face of Transposable Elements: From Harmful Jumping Genes to Major Drivers of Genetic Innovation. Cells. 2021. PMID: 34831175.
The impact of transposable elements in genome evolution and genetic instability and their implications in various diseases. Genomics Inform. 2014. PMID: 25317108.
The impact of transposable elements on gene functionality and genomic structural variations. Genesis. 2020. PMID: 33230956.
The impact of transposable elements on mammalian development. Development. 2016. PMID: 27875251.
The important contribution of transposable elements to phenotypic variation and evolution. Curr Opin Plant Biol. 2022. PMID: 34883307.
The Intricate Evolutionary Balance between Transposable Elements and Their Host: Who Will Kick at Goal and Convert the Next Try? Biology (Basel). 2022. PMID: 35625438.
The Mobilome of Reptiles: Evolution, Structure, and Function. Cytogenet Genome Res. 2019. PMID: 30739120.
The power of “controllers”: Transposon-mediated duplicated genes evolve towards neofunctionalization. J Genet Genomics. 2023. PMID: 37068629.
The role of transposable elements in aging and cancer. Biogerontology. 2023. PMID: 37017895.
The role of transposable elements in health and diseases of the central nervous system. J Neurosci. 2013. PMID: 24198348.
The Role of Transposable Elements of the Human Genome in Neuronal Function and Pathology. Int J Mol Sci. 2022. PMID: 35628657.
The Structural, Functional and Evolutionary Impact of Transposable Elements in Eukaryotes. Genes (Basel). 2021. PMID: 34203645.
Transposable Element Dynamics and Regulation during Zygotic Genome Activation in Mammalian Embryos and Embryonic Stem Cell Model Systems. Stem Cells Int. 2021. PMID: 34691189.
Transposable Element Mediated Innovation in Gene Regulatory Landscapes of Cells: Re-Visiting the “Gene-Battery” Model. Bioessays. 2018. PMID: 29206283.
Transposable Elements and Human Diseases: Mechanisms and Implication in the Response to Environmental Pollutants. Int J Mol Sci. 2022. PMID: 35269693.
Transposable elements and their role in aging. Ageing Res Rev. 2023. PMID: 36773759.
Transposable elements as a potent source of diverse cis-regulatory sequences in mammalian genomes. Philos Trans R Soc Lond B Biol Sci. 2020. PMID: 32075564
Transposable Elements as a Source of Novel Repetitive DNA in the Eukaryote Genome. Cells. 2022. PMID: 36359770.
Transposable elements as essential elements in the control of gene expression. Mob DNA. 2023. PMID: 37596675
Transposable elements as genetic accelerators of evolution: contribution to genome size, gene regulatory network rewiring and morphological innovation. Genes Genet Syst. 2020. PMID: 31932541.
Transposable elements as new players in neurodegenerative diseases. FEBS Lett. 2021. PMID: 34626428.
Transposable Elements Co-Option in Genome Evolution and Gene Regulation. Int J Mol Sci. 2023. PMID: 36768929.
Transposable elements in Drosophila. Mob Genet Elements. 2017. PMID: 28580197.
Transposable Elements in Pluripotent Stem Cells and Human Disease. Front Genet. 2022. PMID: 35719395.
Transposable elements shape the evolution of mammalian development. Nat Rev Genet. 2021. PMID: 34354263.
Transposable elements, genome evolution and transgenerational epigenetic variation. Curr Opin Genet Dev. 2018. PMID: 29525544.
Transposable elements: all mobile, all different, some stress responsive, some adaptive? Curr Opin Genet Dev. 2018. PMID: 29705597.
Transposable elements: genome innovation, chromosome diversity, and centromere conflict. Chromosome Res. 2018. PMID: 29332159.
Transposable Elements: Major Players in Shaping Genomic and Evolutionary Patterns. Cells. 2022. PMID: 35326499.
PubGeneEditingTools
Editing Efficiency Prediction
TnpB Editing Efficiency Predictor (TEEP): https://go.tnpb.app/
Protein Mutational Effect Predictor (ProMEP): https://github.com/wenjiegroup/ProMEP
CRISPR
Chopchop: http://chopchop.cbu.uib.no/
CCTOP: CCTop – CRISPR/Cas9 target online predictor (uni-heidelberg.de)
CRISPR-CAS++ (paris-saclay.fr)
CRISPRloci – CRISPR-Cas annotation (uni-freiburg.de)
crisprSQL – CRISPR off-target database
CRISPR RGEN Tools (rgenome.net)
https://benchling.com/pub/c2c2
http://crispr-analyzer.dkfz.de/
http://www.e-crisp.org/E-CRISP/
http://crispresso.pinellolab.org/submission
http://guides.sanjanalab.org/#/
https://www.crisprindelphi.design/
Addgene: CRISPR Plasmids and Resources
TALEN
Tools | TAL Effector Nucleotide Targeter 2.0 (cornell.edu)
SAPTA TAL Targeter | Tools | tDnA (rice.edu)
ZNF
Zinc Finger Tools (scripps.edu)
The Zinc Finger Consortium | Software Tools (zincfingers.org)